In this second article on microbial identification methods, Accugenix describes methods of tracking and trending organisms at species level and strain-typing in the case of sterility failure or for probiotic health claims.
In industrial settings, such as those for dietary supplement manufacture, strain- typing, or characterisation of micro-organisms, is an important part of the comprehensive environmental monitoring (EM) programme. Of equal importance to the probiotics and nutraceutical industries is the confirmation of specific bacterial strains used for production.
While standard genotypic and proteotypic identification methods increase the ability to identify accurately and consistently and track and trend micro-organisms at the species level, some common microbes cannot be resolved with these approaches alone. Furthermore, strain-typing is the best resource in the case of a major excursion or sterility failure where characterisation to the strain level can be critical.
Subspecies level identification or strain- typing of micro-organisms, as well as discrimination between closely related species, is a challenging goal but necessary since this analysis is very important in investigating a root cause. Some of the common approaches used to differentiate closely related strains compare organisms by considering their genotypic, phenotypic, serological, spatial or temporal characteristics. While the combination of these traits can result in subspecies level identifications, the analysis of multiple characteristics increases the time, labour and expense needed to differentiate isolates, as well as increasing the errors that can arise from qualitative and subjective analyses.
Currently, there are two methods primarily used to differentiate accurately and reproducibly between closely related micro-organisms: ribotyping and single- and multi-locus sequence typing (S/MLST).
Ribotyping is an automated process for characterisation of bacterial isolates and assesses the genetic relationship between strains of the same species. This fragment-based technology utilises restriction enzymes (typically EcoRI and PvuII) to target and cut regions of the ribosomal RNA genes. The DNA fragments are resolved by gel electrophoresis and chemiluminescent probes are used to visualise the banding pattern, resulting in a unique fingerprint of the bacterium.
The pattern or fingerprint is compared with others in a database and assigned to a RiboGroup for characterisation. This fingerprint can be used for tracking at the sub-species or strain level. These patterns can also be generated from different samples at different times. An historical record of the operational environment can be generated and used to determine the level of similarity or differences between the historical isolates and the current isolates as part of an investigation to track the source of contamination.
A more powerful method is emerging in the commercial setting that has increased discrimination at the strain level. This increased resolution is achieved by looking at highly variable single or multiple loci (regions) in the genome. By combining standard genotypic identification methods, such as 16S sequence-based analysis, with multi-locus sequence typing (MLST) or single-locus sequence typing (SLST), it is possible to resolve some of the most difficult organisms to trend and track – for example, in the dietary supplement sector.
A more powerful method is emerging in the commercial setting that has increased discrimination at the strain level
SLST and MLST are well-established, highly accurate sequence-based methods that can be used to distinguish closely-related micro-organisms, providing data that resolves differences or similarities at the subspecies or strain level. Since the foundation of S/MLST is built upon DNA sequencing results, which can be easily catalogued and referenced, these techniques are highly reproducible, unambiguous and scalable. Given the reproducibility of S/MLST between experiments and over time, these methods can be used to determine if isolates recovered from one area are the same as or different from another isolate – a trait that allows for high-resolution trending and tracking projects.
S/MLST methods involve sequencing one to 10 target genes outside the standard regions sequenced for microbial identification (16S, ITS2 or D2). These target genes are known to harbour moderately to highly variable DNA sequences and are protein-coding or housekeeping genes that encode for the proteins necessary for the normal cellular functions of the bacteria. By using the gene sequence, as opposed to the gene product as in enzyme electrophoresis, more variation can be detected resulting in more potential differences per locus.
The addition of multiple loci provides added variation to differentiate further between closely related strains. After sequencing, all the sequences from the multiple gene targets are concatenated (placed end-to-end), aligned and compared with sequences from other organisms.
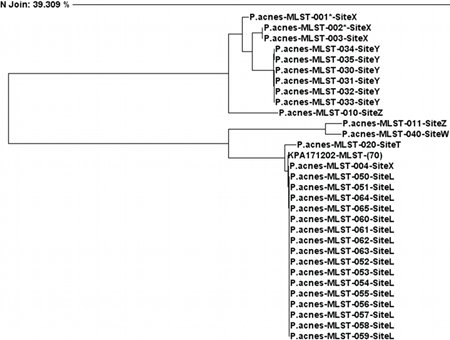
Phylogenetic tree for P. acnes based on MLST combined gene targets
This comparison enables the level of divergence/conservation between micro-organisms to be calculated and displayed with an evolutionary phylogenetic tree. The goal is to determine gene combinations that can give a high level of variability to differentiate to the strain or subspecies level.
The subspecies or strain level identification makes it possible to track definitively the source of contamination or to verify production strains with certainty.
New regulatory guidelines for the manufacturing of dietary supplements, nutraceuticals and other products require testing to determine the microbiological quality of the raw materials, purity of the final product and establishment of a quality programme, assuring that the manufacturing process is sufficiently controlled to prevent contamination or adulteration of the final product and production environment.1-4
Thorough, accurate and reliable identification of the microbial population in the raw materials, final product and manufacturing environment allows for rapid and definitive resolution of sterility failures, alerts and other excursions.
Data from EM sample sites should reflect operational considerations and should be proactively used to create tracking and trending reports on a frequent and routine basis, providing detailed analysis regarding the state of environmental control within the manufacturing area.
Any significant change in microbial flora should be considered in a review of the ongoing monitoring data and used in investigations of excursions to affect the mitigation process and root cause determination.
Using the most accurate microbial identification methods while conducting gridding studies of the manufacturing plant and performing routine screenings allows reoccurring excursions of the same organism to be recognised and identified. Likewise, an increase in the number of micro-organisms recovered in certain areas of the facility may indicate breaches in the HVAC system or other sources of microbial contamination. A recognised shift in the types of organisms recovered from the area may be helpful in locating the source of contamination.
Any significant change in microbial flora should be considered in a review of the ongoing monitoring data and used in investigations of excursions to affect the mitigation process and root cause determination
EM data can be compared against product bioburden data, and the results of the comparison can have an impact on product development, process validation, production and an uninterrupted supply chain. Assurance of product quality through timely management and review of EM trending data can help to maintain a state of control of the manufacturing process and facilities.
Reliable microbial identification systems are required to give consistent and accurate results for tracking and trending. Identification methods that provide inconsistent identifications or no identification for the same isolate are not useful for tracking isolates to their source or for generating trending reports and can lead to misdirected remediation efforts.
Additionally, if a health claim is made for a probiotic, the Latin name (i.e. genus, species and strain designation) of the probiotic micro-organism that is the subject of the claim should be declared.3 Thus, consistent strain-typing is critical for the probiotics industry to assure that the exact strain of the micro-organism in production is established.
DNA sequence tracking
Genotypic methods for identification are based on the rDNA of an organism, which may exhibit but one nucleotide mutation every three million years. The DNA sequence is an unchanging descriptor of the organism and provides a superior tool for tracking and trending. By employing genotypic methods for a monitoring programme, sequences will be obtained for the microbial ecology, and if a name changes due to developments in taxonomy that reclassify an organism, the organism can still be tracked since the sequence will not have changed.
The species name alone may not provide the appropriate evidence to develop a response to a contamination event
If a major excursion occurs, a sterility failure with a product on hold or production that ceases, a thorough characterisation of the microbial population in the manufacturing environment through strain- typing is the preferred method for sourcing the contaminant. Investigative programmes that use only the genus and species name of an organism may not provide enough information to make definitive conclusions in an investigation.
The species name alone may not provide the appropriate evidence to develop a response to a contamination event if there is the potential for multiple strains as sources for that contamination. Many times it is necessary to differentiate microbial flora to the subspecies or strain level to determine definitively the source of contamination. Once a thorough genetic description is generated, it can contribute to determining a root cause, thus allowing remediation of the situation and determination of a corrective action plan in a more timely manner.
Assuring compliance with the regulatory guidelines for microbial monitoring during the production of dietary supplements and probiotics requires accurate identification of the organisms in the microbial environment of the production facility and in the components of the product. Accuracy of identification is key and dependent on the method used to generate and interpret the data as well as the database used as reference.
Genotypic methods are recognised as the gold standard for identification and strain- typing, but the novel mass spectrometry-based technology provides a sound alternative for routine monitoring with accurate bacterial identification to the species level. When these consistent, qualified methods are combined with relevant, up-to-date, validated libraries, identification of organisms from the manufacturing environment to the species or strain level is unsurpassed.
By using dependable methods to obtain identifications, you can be certain that tracking the organisms in your environment, or the organisms that are your product, will be accurate and consistent and aid in documenting control of the production environment and leading to overall brand protection and consumer confidence.
References
1. FDA Regulation 21 CFR Part 111 – Current Good Manufacturing Practice in Manufacturing, Packaging, Labeling, or Holding Operations for Dietary Supplements (2011).
2. Dietary Supplement Health and Education Act of 1994.
3. FAO/WHO Joint Working Group Meeting, Guidelines for the Evaluation of Probiotics in Food (2002).
4. Bacterial Library Listing and Comparison. White Paper, Accugenix (2011).
This two-part article is based on a technical note published by Accugenix. The first part can be read here.