The process of wearing, laundering and sterilising reusable cleanroom garments can impact their physical properties and change garment functionality. Laundering and wear abrade garment fibres. Simultaneously, changes to the polymers that make up the garments can occur at the molecular level. Although routine visual inspection is often part of garment quality evaluation programmes, non-visible properties also change with time.
When selecting reusable garments for use in cleanroom environments, it is important to understand how they will perform over their intended life cycle. Consideration of these properties should be part of the decision process for when to take reusable garments out of service.
Physical property data are often available for new cleanroom garments; however, there are less data available throughout the entire garment life cycle. To aid in garment choice, DuPont conducted a study of the physical properties of reusable cleanroom garments after a set number of laundering and gamma radiation exposure (sterilisation) cycles.
The results are outlined here.
Methodology: Two sets of commercially branded, reusable coveralls were purchased for testing1 and designated as Garment A and Garment B. Garments were made of woven polyester with integral carbon fibre for electrostatic decay properties. Garments were laundered2 and subsequently exposed to gamma radiation; this was considered one cycle. This process was repeated for 30 cycles. Garments were removed for testing after pre-determined numbers of cycles (see Figure 1).
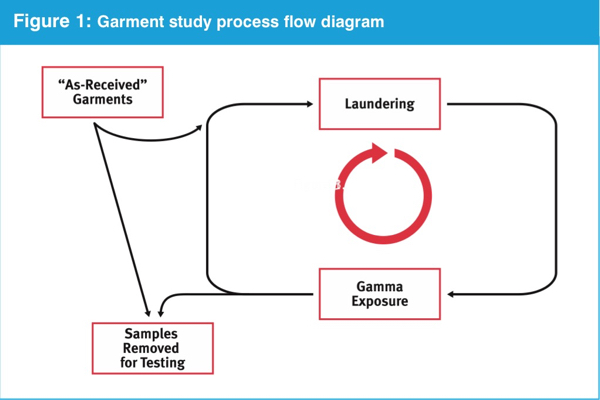
Laundering and gamma exposure were conducted through 30 cycles
Not all properties were tested at the same frequency. Initial properties of the garments were either measured on “as-received” garments or garments that had been laundered one time, but not exposed to gamma radiation. Parameters for garment laundering and gamma exposure were consistent throughout the study.
Garments were not worn or exposed to simulated work scenarios between cycles and the effect of routine garment “wear and tear” was not part of this study.
A summary of the garment testing methods is shown in Table I. Testing was done at third-party laboratories.4 Results for property testing are shown with the average and the Bonferroni confidence interval on the mean. Changes in both absolute performance and variability within the garment population may factor into formulation of end-of-life criteria.
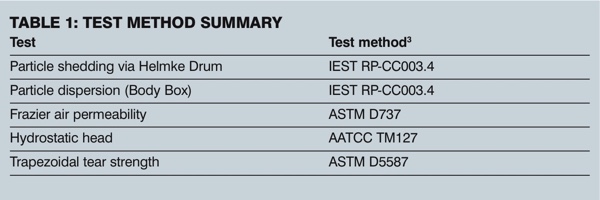
Certificates of processing (COP) for each gamma radiation exposure were received. Dose range per cycle was target minimum of 25kGy and target maximum of 40kGy. Received dose was calculated by summing the minimum received and maximum received doses per cycle, as indicated on the COPs. Mid-dose was calculated by averaging minimum and maximum dose per cycle (Figure 2).
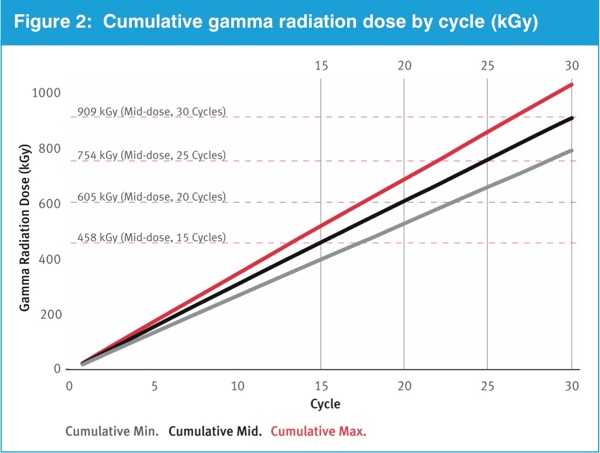
Chart of cumulative minimum, maximum and mid radiation dose as a function of exposure cycle. Mid-dose was calculated by averaging minimum and maximum dose
Results and discussion
Properties related to protection, durability and comfort are shown below to indicate trends in garment and fabric performance after laundering and exposure to gamma radiation.
Radiation dose and polymer molecular weight: The impact of gamma radiation exposure on a variety of polymers is well studied.5 Although multiple reaction mechanisms can occur simultaneously, there is typically a predominating reaction type. The extent and type of each reaction depend on many factors and combinations of factors, including:
- Polymer composition (different polymers behave differently)
- Presence or absence of air during irradiation
- Crystallinity of the polymer and changes in crystallinity
- Physical configuration (e.g., fibre, film or tubing)
- Additional processing (e.g., laundering, calendering or surface treatment)
- Presence of antioxidants or other additives in the polymers
- Cumulative radiation dose
The two primary reaction mechanisms that occur in polyester (PET) after exposure to gamma radiation are chain scission and cross linking.6,7 Changes in the polymer makeup can result in changes to a garment’s physical properties. To better understand which mechanism predominated under the conditions of this study, PET molecular weight was measured by size exclusion chromatography (SEC) using hexa-fluoro-isopropanol (HFIP) as the solvent.8
Results for Garments A and B overlapped, so the data were grouped (Figure 3). Because the molecular weight of the PET decreased with laundering and exposure to gamma radiation, chain scission was the predominant mechanism. Because garments were both laundered and exposed to gamma radiation, these data include the simultaneous impact of both factors.9
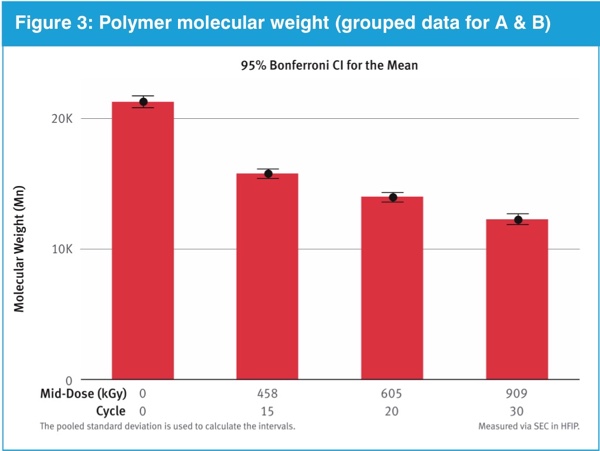
Number average polymer molecular weight (Daltons) for garments A and B
Physical properties: Garment physical properties can be grouped into several categories: those related to protection, those related to durability and those related to comfort.
Protection properties: The primary function of cleanroom garments is to protect a product or a process but in some instances garments also protect the worker from hazards. To represent process protection, particle shedding was measured via the Helmke Drum method (Figure 4), and particle dispersion via the Body Box method (Figure 5). To represent worker protection against incidental, aqueous splash, hydrostatic head was evaluated (Figure 6).
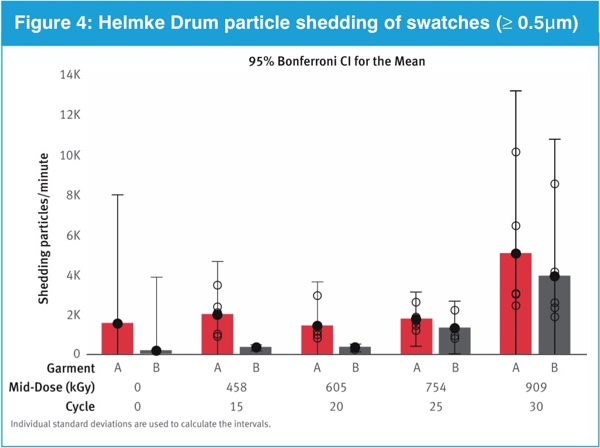
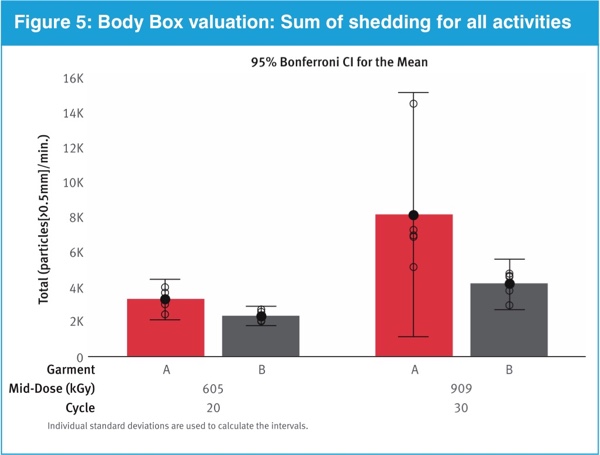
Body Box testing — sum of total particles/minute shedding rate for all
activities specified in the method
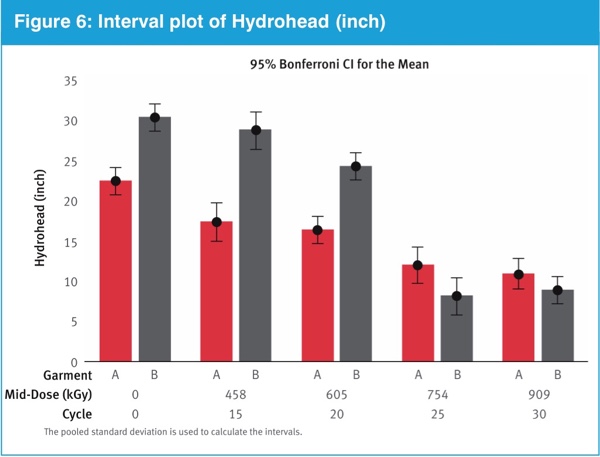
Hydrostatic head
During the course of testing, neither Garment A nor Garment B tumbled uniformly in the Helmke Drum. Both became tangled in the test apparatus. To minimise variability in fibre-shedding results due to non-uniform garment tumbling, swatches measuring 20 x 30cm were cut from the test garments and tumbled instead.
Because of the impact of cut edges on shedding behaviour, particle shedding data based on swatches cannot be extrapolated to whole garment performance.10 However, swatch data can be evaluated for performance trends. The data shows that particle shedding increased after 25 cycles or exposure to cumulative mid-dose of 754kGy, but was fairly consistent until that point.
Body Box testing measures not only particle generation from the garment, but can also indicate its function as a particle barrier. In this test, a fully garbed test subject conducts a series of movements inside a box supplied with HEPA-filtered air. Air in the box is sampled by a particle counter and shedding rate is reported as a function of activity as well as a total rate for all activities11 conducted during the test. This data also showed a shift in performance after increased cycles of laundering and gamma radiation exposure (Figure 5).
Both the Helmke and Body Box data show an increase in both amount and variability of shedding.12 Cleanroom operators who are sensitive to particle shedding should consider establishing a monitoring programme to determine when garment performance no longer meets use requirements. Particle sizes typically monitored in a cleanroom are too small to be visible to the naked eye, so visual inspection alone will not necessarily indicate an increase in garment shedding.
Hydrostatic head was used to evaluate fabric performance against an aqueous challenge. Fabric was subjected to a water column of increasing pressure until three drops penetrated the fabric. The data show a drop in performance as a function of exposure to laundering and gamma radiation. If garments are considered for incidental, light aqueous splash protection, understanding the use requirements per cycle is important.13
Durability: Durability is another aspect of garment performance. Garments should withstand normal “wear and tear.” Without adequate durability, garment breach is possible. To understand the impact of laundering and exposure to gamma radiation on garment durability, trapezoidal tear strength was measured (Figures 7 and 8). Cross direction (CD) tear strength is shown in Figure 7 while machine direction (MD) tear strength is shown in Figure 8.14 Often in woven garments, there are different constructions in the two directions, so differences in tear values between MD and CD are expected.
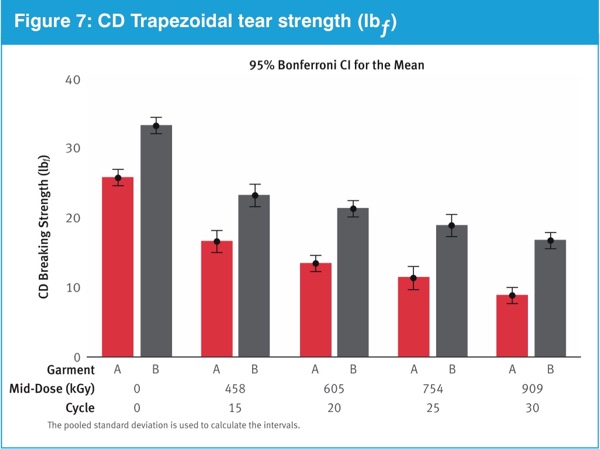
Cross Direction (CD) trapezoidal tear strength
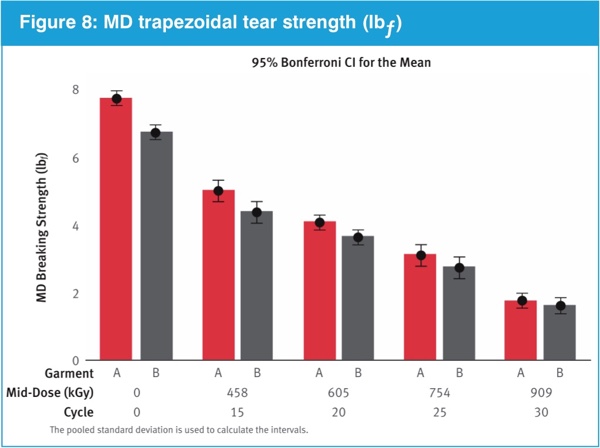
Machine Direction (MD) trapezoidal tear strength
Testing showed that garment durability decreases with increasing cycles of laundering and exposure to gamma radiation. Reducing potential impact from garment tearing is important, especially in cleanrooms and controlled environments where workers may have physical activities such as climbing stairs or bending to monitor or adjust equipment.
Comfort: Garment performance related to worker comfort was also evaluated. Though assessment of garment comfort encompasses a wide variety of fabric test methods, air permeability provides information on air movement through a garment. Frazier air permeability is shown in Figure 9.15
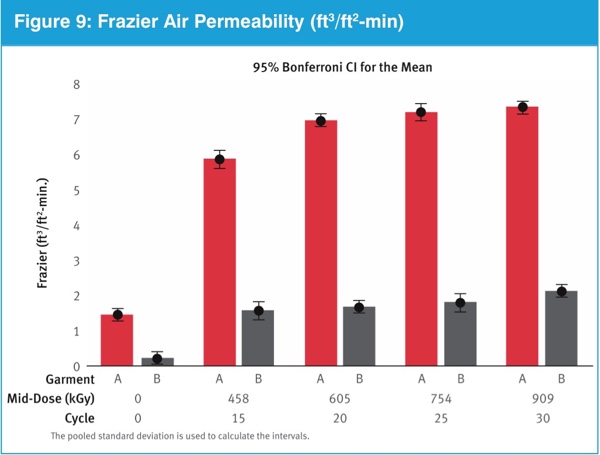
Frazier Air Permeability (ft3/ft2-min.)
The results show increasing air permeability with increasing exposure to laundering and gamma radiation. This can be contrasted with the decrease in hydrostatic head performance previously described.
Worker comfort is an important feature in garment assessment; however, it is important to understand the trade-off between air permeability and barrier. Is a garment with increased air permeability still providing the required barrier performance?
Key takeaways
The data outlined here demonstrate that garment properties do change after a number of laundering and gamma exposure cycles. These changes are not always visible to the naked eye, so visual garment inspection alone may not be sufficient to understand garment performance. Based on these findings, the following guidelines are recommended:
- Consider performance data over the entire garment life cycle
- Enact testing protocols to monitor the performance of garments as they age, based on the risk assessments and needs of each individual cleanroom
- Establish criteria for taking garments out of service when they no longer meet functionality requirements
It should be noted that since garment requirements vary by cleanroom operation, establishing initial and ongoing fitness for use is the responsibility of the end user. Garment assessment may require evaluation of additional information beyond what is presented here. For example, seams and closures may have lower barrier properties than fabric. This difference was not specifically assessed in this study. Properties of garments and fabrics subjected to other conditions, including different sterilisation methods, may also vary.
Footnotes
- Two different production lots of each garment type were obtained. To track garments through the study, each one was given a unique ID number. For each test, multiple garments from each production lot were part of the test population.
- Garments were laundered in a commercial facility, under settings typically used to process similar cleanroom garments.
- IEST = Institute of Environmental Sciences and Technology. ASTM = American Society for Testing and Materials. AATCC = American Association of Textile Colorists and Chemists.
- Body box, Helmke Drum, trapezoidal tear. Frazier and hydrohead tests were conducted in DuPont laboratories.
- Skiens, W. E. Radiat. Phys. Chem., 1980, 15, p47-57.
- Potnis, S. P., Shetty, S. M., Rao, K. N. and Prakash, J. Die Angew. Markromol. Chemie, 1969, 6, p127-135.
- Nair, P. D., Sreenivasan, K., and Jayabalan, M. Biomaterials, 1988, 9, p335-338.
- Measured at the DuPont Corporate Center for Analytical Services.
- The impact of only laundering the garments was not studied simultaneously to determine the relative impact of one process step versus the other.
- Per the method, a categorical rating is typically assigned to full garment performance based on shedding rate. Helmke Category I garments are the lowest shedding rate.
- Data for each individual activity in the testing is not shown here.
- For these figures, individual data points are shown to demonstrate increased variability. Individual data points are omitted from other figures for clarity. Note that Helmke drum result for 0 cycle garment has less data than other cycles, so calculated confidence intervals are larger.
- Evaluation of garment performance for protection against hazardous or non-aqueous liquids requires testing via additional methods. Higher numbers in the hydrostatic head test indicate higher pressure at the penetration of three drops.
- In this test method, CD direction testing tears MD direction fibres and vice versa. Higher numbers indicate stronger fabric.
- Higher numbers indicate increased air flow.