The story told in Figure 1 is commonplace for many cleanroom facilities around the globe. The rise in energy prices brought on by the COVID-19 pandemic, amongst other significant geopolitical events, has once again emphasised the need to explore energy efficiency measures for all cleanroom operating organisations, such as those within pharmaceutical and biotech manufacturing.
But for these companies, there is an even greater motivation to tackle energy intensity. In 2016, the United Nations Sustainable Development Goals (SDGs) were announced. These goals have sought to establish a framework for which matters of global significance, such as economic stability and environmental sustainability, can be addressed. Of relevance to life science, SDG 3 promotes well-being and looks to ensure healthy lives. Goal 9.4 aims to upgrade industries and infrastructure to make them more sustainable, while 12.2 looks at the sustainable management and efficient use of resources.
With this in mind, the majority of the top 20 global life science manufacturers have since made public commitments to improve their sustainability, with many setting near-term net zero or zero carbon objectives due within the next two decades. For the likes of GSK and AstraZeneca amongst others, these targets have been verified by the Science Based Targets initiative (SBTi), confirming that their successful delivery will help to limit global temperature rise to 1.5°C.
Yet this subset of net zero committed companies is not representative of the wider pharmaceutical and biotech industry, with the latest My Green Lab industry report concluding, "While the largest companies are leading with ambitious carbon targets … the rest of the industry is lagging.
Of the 75 companies in our dataset … only 9% have targets aligned with a 1.5°C warming scenario by 2030”. There is a stark contrast between the world’s largest life science businesses and the often smaller contract manufacturers that constitute their supply chain. This is indicative of a wider issue. Scope 3 emissions (indirect emissions within the value chain) are 4.3 times higher than combined scope 1 (direct emissions from owned sources) and scope 2 emissions (indirect emissions associated with purchased energy or steam) for companies in the pharmaceutical industry, suggesting a highly carbon-intensive value chain which will require aggressive action in order for the world’s largest life science companies to achieve the scope 3 reduction targets.
Through the Carbon Disclosure Programme (CDP), which assists businesses to understand their carbon footprint, the largest pharma and biotech businesses are beginning to get to grips with their value chain emissions, asking their suppliers to disclose their own carbon performance. For some CDMOs, this means answering tough questions about their operations. Being a sustainable-mind supplier is now a competitive advantage – as the top 20 life science organisations begin to factor sustainability into their supplier selection process.
Sustainability enhancement in cleanrooms
To reach these sustainable aspirations, the role of the cleanroom must be considered. For the life science industry, cleanrooms serve an essential function – a sterile space within which highly sensitive products and formulations can be produced and packaged under controlled conditions. In doing so, the safety of the product and thus the patient is ensured. In creating the conditions within which this production can occur, cleanroom facilities consume significant amounts of energy with research citing cleanroom facilities as consuming 30-50 times the energy of an average US commercial building. This energy intensity stands as an obstacle for any life science organisation aiming to achieve net zero operations.
One possible avenue to tackle cleanroom energy performance is that of cleanroom airflow reduction, which serves as a great opportunity for sizeable carbon (and also cost) optimisation. Airflow reduction refers to the act of decreasing the rate at which air within the cleanroom space is actively replaced. Commonly, the phrase “air change rate” (ACR) is used as a convenient metric for contextualising cleanroom airflow into an easily interpretable number. As an example, it is not unusual to find pharmaceutical Grade C cleanrooms operating anywhere between 20 to 60 air changes per hour (ACPH), although occasionally, this figure can be even higher.
We know that airflow reduction is a substantial opportunity for carbon and operational cost reduction due to the fan affinity laws, which state that fan power is related to airflow by a “cube” law, meaning a 10% reduction in flow can result in a 28% reduction in fan power consumption. This approach to improving energy performance becomes even more appealing for cost-conscious businesses when we consider that 65-75% of cleanroom costs are typically associated with energy consumption. Of course, prior to simply reducing the supply of air into a space, there are a number of factors that must be considered, with some of these explored in the passage below.
Gowning
The importance of gowning in controlled environments cannot be overstated. When testing the effect of cleanroom grade C clothing at airflow rates as low as 5 ACPH, our experimentation found that in-operation particle counts remained within acceptable levels of environmental control, and even within grade B requirements – but the corresponding microbiological results demonstrated less effective environmental performance, with levels not compliant for grade B conditions. Further investigation found that increasing the airflow rate had little impact on improving microbiological performance.
For good control of viable particles, it appears that gowning is a more essential factor than the amount of air being introduced to the space, as operators are a greater source of biological contamination than the supporting heating ventilation and air conditioning (HVAC) system.
The results of this experiment call into question the importance of air change rates as a critical control parameter and imply that the airflow rate is not necessarily a reliable indicator of cleanroom contamination performance.
Regulatory standards
Historically, HVAC systems that supply cleanrooms have at times been used to compensate for, or even mask poor process containment, whether that is in relation to the abatement of heat gain or the removal of particles introduced via the process - such as in tablet pressing, for example. In facilities where there are difficulties in process containment, reducing the ACPH could exacerbate these challenges. Due to the variability of effective process containment in the industry and indeed the range of processes taking place from one facility to another, ISO 14644-16 encourages a risk-based approach for calculating ACPH. In alignment with this, no international regulatory body has enforced specific numbers for ACPH (although some pose recommendations), which would further add weight to the notion that a risk-based calculation of cleanroom airflow is the favourable approach to take.
Yet for many life science organisations, this has not been implemented. The minimum number of air changes per hour in a cleanroom space is still specified within internal quality documentation. In our experience across hundreds of pharmaceutical sites, many facilities sit well above the minimum threshold for internal compliance, thereby missing a feasible opportunity to significantly reduce their site’s energy bill and carbon footprint while remaining in line with organisational parameters for operation.
Dynamic cleanroom control
Ultimately, cleanroom airflow rates that are tethered to a fixed value neither provide the lowest energy performance possible nor guarantee a compliant environment at all times, owing to their inability to adapt to the contamination challenge present in the space. Even when employing an overnight setback of the ACR, at a time when little to no cleanroom activity is expected, there is still no absolute guarantee that the space is perpetually within the compliance limit for effective contamination control.
Utilising live contamination data, alongside other critical parameters (pressure, temperature, humidity etc.), it is possible to adapt the airflow (and therefore ACPH) to match the demand from the space continuously, resulting in a system capable of reacting to the present contamination challenge and controlling this well within GMP compliance limits - whilst achieving more significant energy reduction than would ever be attempted via static airflow reduction.
Data from the world’s first life science installation of an Intelligent Cleanroom Control System (ICCS trademarked) at the Cambridge Pharma facility in the UK has highlighted the benefits of this dynamic control. As can be seen in Figure 2, the system utilises a complex algorithm to analyse particle generation rates and thus modulates the airflow before the rising particle concentration can pose any risk to the compliance limit or product safety. In doing this, the system is able to proactively maintain performance well below the particle concentration set point (i.e. 10% of the classification limit) at all times. It should be noted that this control limit is an adjustable variable and is dependent on the user's requirements.
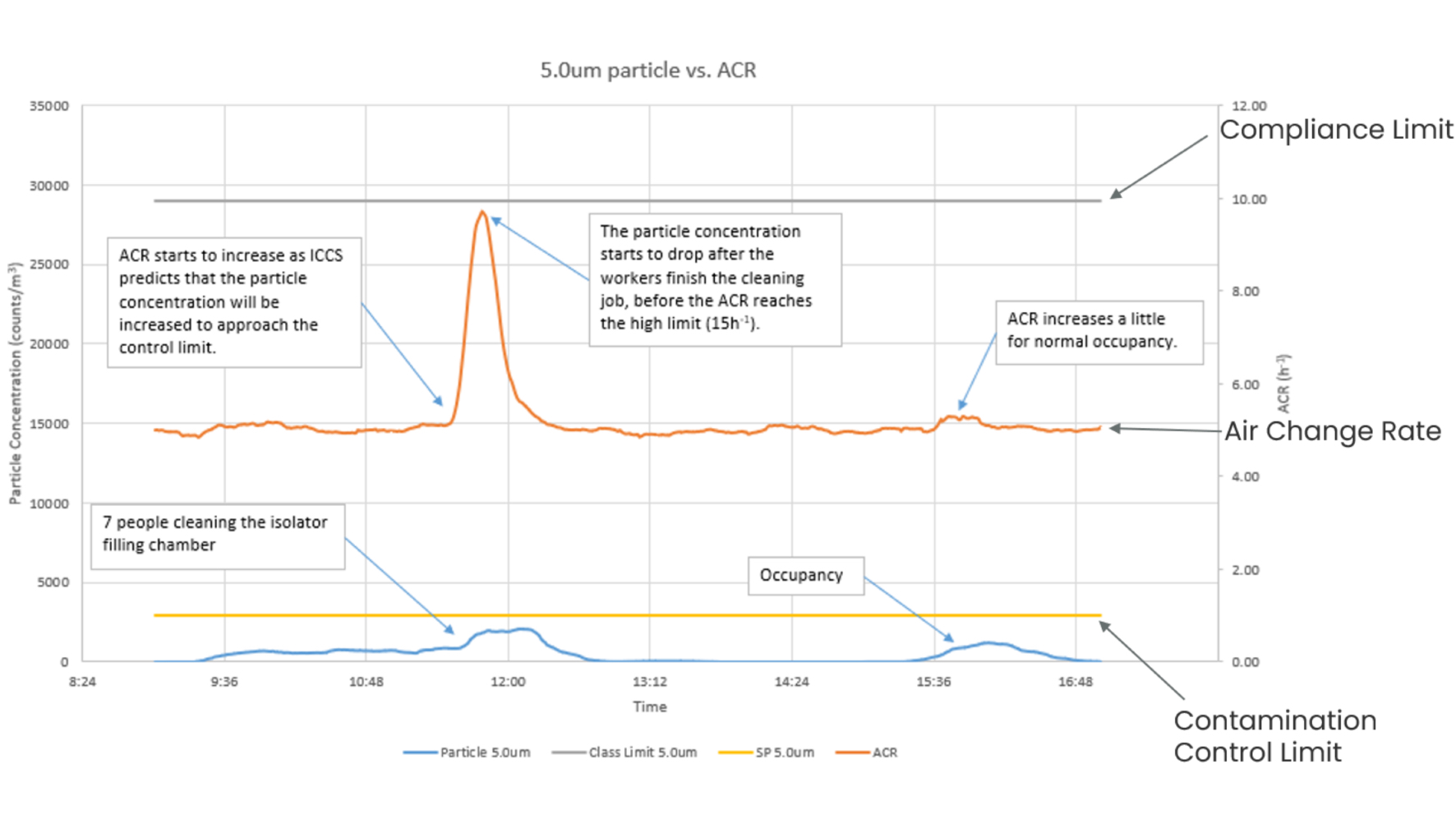
Exploring the data further (Figure 2), when the particle generation rates do increase as several operators enter the environment to perform cleaning routines, the airflow rate also increases rapidly - going from 5 ACPH to peaking at 9 ACPH and then quickly returning to the typical value of 5 air changes per hour.
As a result of the dynamic system in place, it is possible to maintain the ACR at around 5 ACPH most of the time. This is significantly lower than the aforementioned 20 to 60 range that is frequently found in Grade C spaces, thereby saving a significant quantity (80%) of energy when compared with typical industry cleanrooms, per m2 (Figure 3).
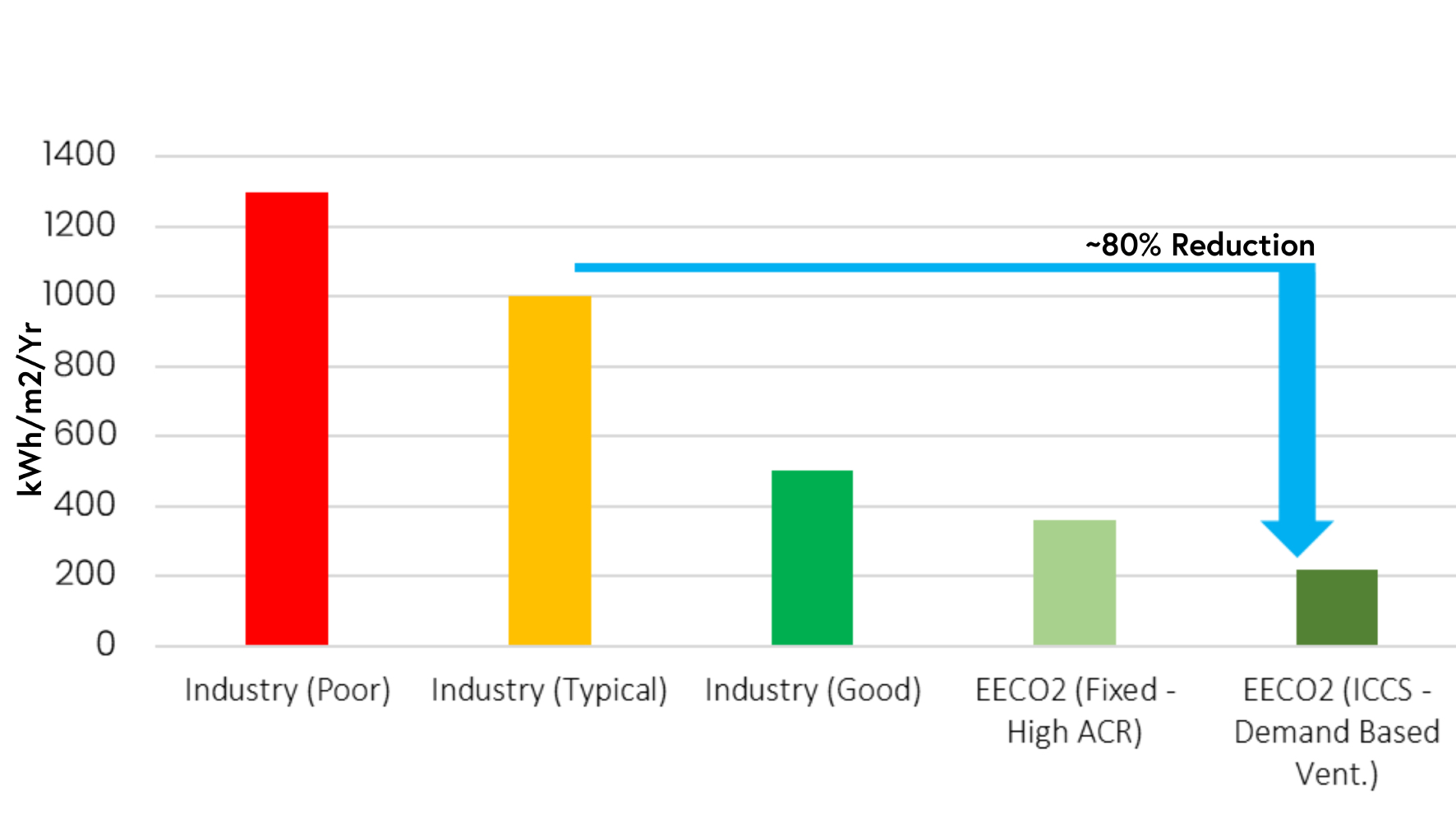
But non-viable particles are not the only contamination factor that is important in cleanroom spaces. Viable particulates pose a serious risk to product safety and must also be closely monitored and controlled to avoid exceeding the requirements outlined in Annex 1. The data from the Cambridge Pharma micro testing supports this (Figure 4) - even operating at very low air changes per hour, the facility is demonstrating good control of micro-biological contamination thanks in large part to a successful cleaning and gowning regime.
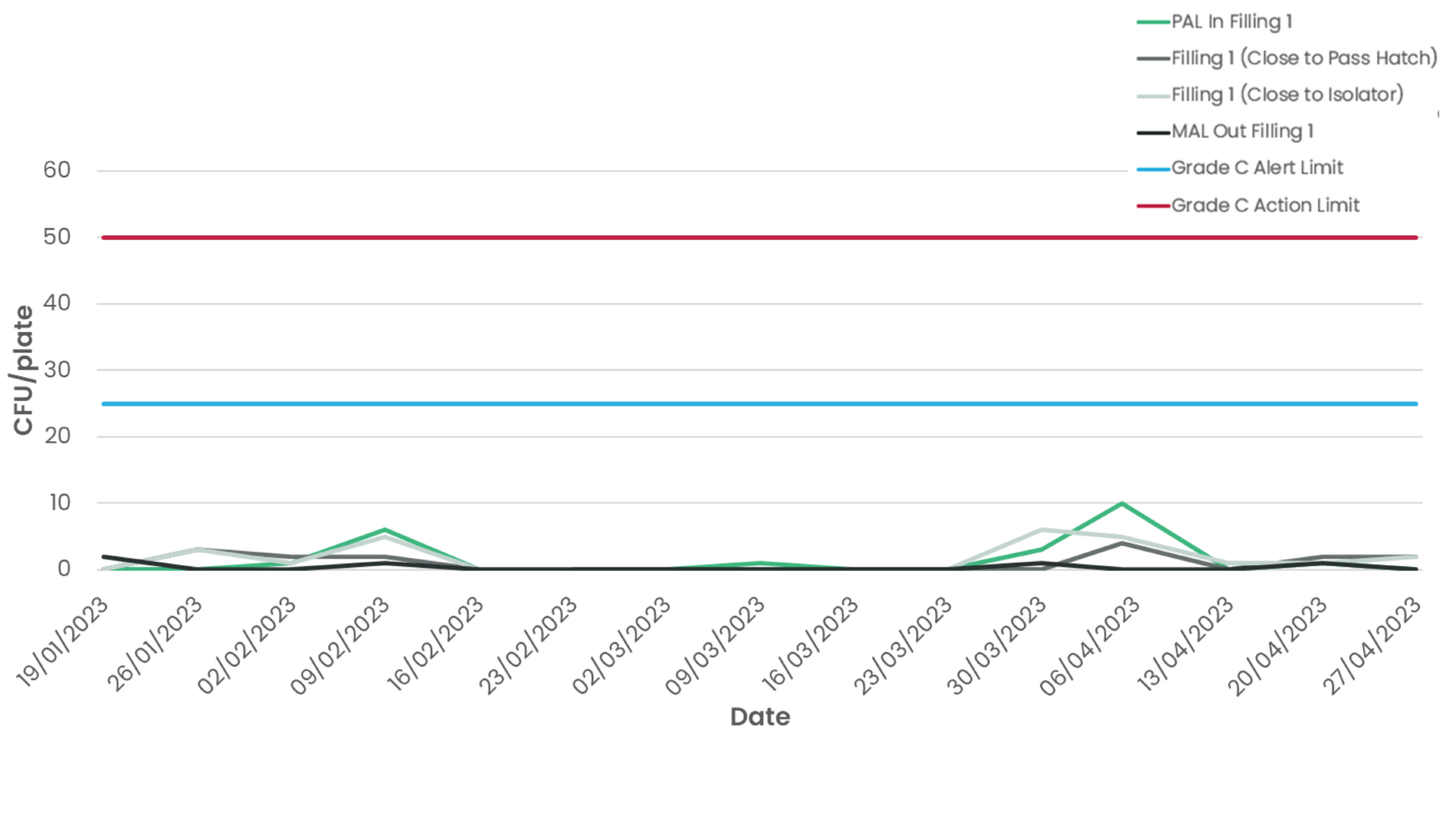
Figure 4 – Micro-biological data from Cambridge Pharma supports the feasibility of dynamic cleanroom control from a contamination standpoint.
Quality-driven approaches for improving sustainability performance, such as dynamic control in cleanrooms, are a big piece of the net zero puzzle. The increasing demand for sustainable operations, accelerated by internal decarbonisation targets and customer requirements, will drive many life science companies to embrace new ways of decarbonising their operations. The significance of this extends beyond the realms of improving the sustainability of individual medicines but ultimately translates into improved health across the globe, which for all patient-centric life science organisations, is a future worth aiming for.