It is an unfortunate fact of modern economic life that energy costs are rising steadily while building management budgets are static or even declining. For new buildings it is fairly easy to incorporate the latest energy-saving systems and materials, but existing facilities may be plagued by inadequate or non-functioning systems that make the search for technologies to increase energy efficiency particularly pressing.
The Hutchison MRC Research Centre opened in March 2001 as a joint venture between the UK’s Medical Research Council (MRC) and the University of Cambridge to address a need in translational cancer research. It is a standard biomedical ‘wet lab’ occupied by 150 scientists and support staff. The facility was built and operated by MRC and supported by the MRC regional estates team.
On 1 October 2013 it was transferred into the University of Cambridge and is now staffed by University employees and supported by the University of Cambridge team. It consists of five floors, three of which house the laboratories where the research is carried out, one support floor and a plant room on the top floor.
The laboratory floors are arranged into four large lab areas with offices in the corners divided by a cross-shaped area in the centre that houses the equipment rooms and cold rooms (see Figure 1).
According to Research Centre Manager Brian Richardson, the building was constructed on a very tight budget and there were several design flaws in the area of heating and ventilation. When the bills started to come in, he realised he had to do something to address energy consumption, both in terms of the way the building functions and how it is used by its occupants.
An energy-saving initiative was put in place to encourage recycling, reminding people to turn off lights and equipment, and purchasing whenever possible the most energy-efficient equipment available.
‘We also established a Green Team – getting support from like-minded individuals in the building to influence colleagues and put forward ideas,’ Richardson recalls. The aim was to make people realise that if the bills remained at current levels there would be less money available for science and research.
The aim was to make people realise that if the bills remained at current levels there would be less money available for science and research
‘That was all very well, but although it was improving people’s behaviour, I’m not sure it was actually improving our bottom line,’ he says. So he then turned his attention to the building, looking at what could be improved, what could be removed and what could be utilised better in terms of heating, cooling, lighting, water, gas and electricity.
Richardson is the Research Centre Manager, but facilities management is not his area of expertise, so he turned to similar organisations, specifically the University of Cambridge, which has extensive initiatives across various departments. He also consulted the MRC maintenance team for help and advice.
‘We used our own knowledge of month-on-month running of this building as an opportunity to deal with some of the things that had been irritating about it for so many years, on the basis of improving environmental efficiency,’ says the MRC maintenance team’s David Robinson. ‘That is at the heart of energy-saving principles – before going for large capital projects, it is essential to understand how the building itself works first.
‘There are many things that you can do to your plant and systems that save significantly and make the building more comfortable.’
Before going for large capital projects, it is essential to understand how the building itself works first
It is estimated that heating, ventilation and air control (HVAC) systems account for around 40% of energy consumption in laboratories, so this was the first area to come under scrutiny. For example, the valves that controlled the chilled water heat exchangers in the air handling units where the air comes into the building were leaking, says Robinson, so water was being chilled even when it was not required, and then reheated to the correct temperature.
There were also humidity blankets in the air handling units that had not only not worked for years, but they were also creating pressure differential with a consequent high demand for energy with no purpose. Removing these from the air handling units eliminated the pressure differential, resulting in a drop in the fan curve and a fall in energy demand.
The inability of the building’s structured air handling system to meet demand had also required the installation of standalone fan coil units in the offices to maintain comfortable temperatures all year round as well as to keep the equipment rooms cool. But because they were retrofitted, the units ended up fighting the structured system with wide temperature fluctuations throughout working hours.
As a trial, Robinson linked several of the fan coil units and restricted the ability of the users to alter the ambient temperature to just a few degrees. This meant that the rooms could be made to reach set point by the time employees arrived in the morning and would remain at a comfortable temperature throughout the day.
By far the most capital intensive part of the project was the retrofitting of a laboratory ventilation management system from Aircuity, the smart airside efficiency company that provides building owners with sustained energy savings through its intelligent measurement solutions. Rather than the ventilation system running constantly 24 hours a day, 365 days a year at a rate of 20 air changes an hour, the Aircuity system organises the ventilation in accordance with the rate of occupancy of the laboratories.
We are now using our energy efficiently at the point where it is actually needed
‘If nothing is happening in the space, the air change rate for that space is minimal. If there is something going on then the air change rate is ramped up to accommodate,’ Robinson explains. ‘So if you have two people in the building working in one lab at three o'clock in the morning it is only that space that is fully ventilated.
‘If there are labs that are not being used at any time, the ventilation will drop down to tick-over rate – it isn’t time-based, it works on demand. We are now using our energy efficiently at the point where it is actually needed.’
The three drivers behind ventilation within a laboratory are: hood flows, thermal load requirements and dilution requirements. It is on the last of these where Aircuity focuses its attention.
Probes operated by solenoids are located in the ductwork of the MRC labs and take a sample of the air. Each sensor can handle a lab with an area of approximately 100–120m2. The probes are linked by a structured cable with a central tube at its core that takes the packets of air to a sensing suite for analysis; around the tube are located the power and command signal cables, all bound in an outer wrap. To avoid the risk of contaminants collecting inside the tube that could influence the next reading, a nanotech carbon net or web is extruded all through the tube so that nothing can get a charge and cling to the side of the tubing.
This structured cable is relatively rigid, making the retrofit into a building that was already up and running fairly easy with minimal disruption for users. From the sensing points in the lab, the cable goes via a router, which can handle up to four zones – i.e. an entire floor of labs – to the sensor suite.
Because the MRC building is not particularly large, each lab is sampled every eight minutes; in a larger installation the interval would be greater, but there would never be more than 15 minutes between samplings. Data collected by Aircuity suggests that is the optimum for adjusting the airflow rates in the lab and keeping it safe.
The room temperature is measured at the location of the probe, while the dew point is measured at the sensing suite, enabling the humidity in the lab to be calculated. The system also measures carbon monoxide, carbon dioxide, particle count and total VOCs.
According to the analysis, the sensor suite then instructs the laboratory’s variable air volume (VAV) system to reduce or increase the airflow volume rates. The MRC building was set up originally to have air change rates of 18–20 an hour but is currently running at between two and four changes an hour.
Although it is intended first and foremost as an energy-saving solution, the system also provides a large amount of information about environmental health and safety
The system is effective, accurate and low maintenance. And although it is intended first and foremost as an energy-saving solution, the Aircuity system also provides a large amount of information about environmental health and safety (EH&S). It is no substitute for safe practice, and works best in an environment where those practices are already in place and being adhered to, but by monitoring and reporting in real time it can highlight trends or pinpoint areas of concern that may need to be addressed. By analysing the data, for example, it may be possible to identify processes that should be moved into isolators or fume cupboards.
Concerns that reducing the air change rate in the labs could compromise worker safety in case of a spillage had to be allayed, so the health and safety team at MRC was involved throughout the implementation of the system.
Essentially the system is failsafe: if the sensors detect anything in the atmosphere that should not be there, the system ramps up to full ventilation. Similarly, if any part of the sensor system were to fail, full ventilation would also be initiated. In the worst-case scenario in the MRC building there would be an interval of less than eight minutes before any spillage was detected.
There are three aspects to the Aircuity system:
1. The hardware (OptiNet System), which is the enabling technology consisting of the cabling and the sensor suites.
2. The Advisor services that use the data gathered by the OptiNet system and analyse it to assess how the building is performing; the information is displayed on a dashboard and communicated through summary reports, smart notifications and data graphing and exporting.
3. The Assurance Services, which include ongoing maintenance and recalibration of the system. These include a six-month swap-out of the sensors with newly calibrated sensors. The old ones are shipped back to Aircuity for testing, refurbishment, recalibration and reuse, which keeps maintenance costs down. There is also remote monitoring of the system with diagnostics to make sure all the components are functioning.
4. Last of all, there is an ongoing warranty on many of the components in the system, including the information management server and the vacuum pumps.
The Aircuity system has been up and running since May 2013 so it is early days to gauge its overall effectiveness. It is also impossible to say what proportion of any savings can be attributed to the Aircuity system since it was implemented in tandem with the other energy-saving initiative. Other factors also need to be taken into account, such as the rising occupancy of the building and the exceptionally hot weather last summer.
Nonetheless, Richardson saw a fall of 37% in gas consumption in kWh in the period May to October 2013 compared with the same months the previous year (see Figure 2). Electricity consumption fell by only 3% in the months from May to September, which he admits was disappointing but may be due to higher demand on the chillers and fans because of the heatwave.
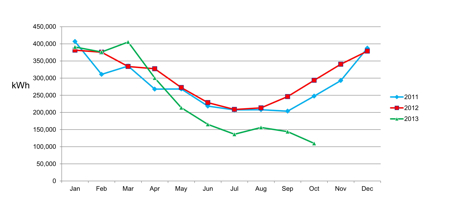
Figure 2: Comparative energy consumption at the Hutchison MRC Research Centre
A longer-term analysis is necessary, but in the meantime the £20,000 reduction in gas costs is very welcome. ‘We are paying our own energy bills, so that is directly affecting my budget,’ he points out. ‘If I can’t pay my bills it will have to come out of the science department.
‘It takes time to properly interrogate the Aircuity system and make the most of what it is capable of doing.’